Executive Summary
A dozen U.S. states, from California to New York, have joined dozens of countries, from Ireland to Spain, with plans to ban the sale of new cars with an internal combustion engine (ICE), many prohibitions taking effect within a decade. Meanwhile, the U.S. Environmental Protection Agency (EPA), in a feat of regulatory legerdemain, has proposed tailpipe emissions rules that would effectively force automakers to shift to producing mainly electric vehicles (EVs) by 2032.
This is all to ensure that so-called zero-emission EVs play a central role in radically cutting carbon dioxide (CO2) emissions. To ensure compliance with ICE prohibitions and soften the economic impacts, policymakers are deploying lavish subsidies for manufacturers and consumers. Enthusiasts claim that EVs already have achieved economic and operational parity, if not superiority, with automobiles and trucks fueled by petroleum, so the bans and subsidies merely accelerate what they believe is an inevitable transition.
It is certainly true that EVs are practical and appealing for many drivers. Even without subsidies or mandates, millions more will be purchased by consumers, if mainly by wealthy ones. But the facts reveal a fatal flaw in the core motives for the prohibitions and mandates. As this report illustrates:
- No one knows how much, if at all, CO2 emissions will decline as EV use rises. Every claim for EVs reducing emissions is a rough estimate or an outright guess based on averages, approximations, or aspirations. The variables and uncertainties in emissions from energy-intensive mining and processing of minerals used to make EV batteries are a big wild card in the emissions calculus. Those emissions substantially offset reductions from avoiding gasoline and, as the demand for battery minerals explodes, the net reductions will shrink, may vanish, and could even lead to a net increase in emissions. Similar emissions uncertainties are associated with producing the power for EV charging stations.
- No one knows when or whether EVs will reach economic parity with the cars that most people drive. An EV’s higher price is dominated by the costs of the critical materials that are needed to build it and is thus dependent on guesses about the future of mining and minerals industries, which are mainly in foreign countries. The facts also show that, for the majority of drivers, there’s no visibility for when, if ever, EVs will reach parity in cost and fueling convenience, regardless of subsidies.
Ultimately, if implemented, bans on conventionally powered vehicles will lead to draconian impediments to affordable and convenient driving and a massive misallocation of capital in the world’s $4 trillion automotive industry.
Introduction: Reactionary Revolutions
Few doubt, even if some lament, the centrality of the automobile in modern society. As the late MIT historian Leo Marx put it: “To speak, as people often do, of the ‘impact’ of . . . the automobile upon society makes little more sense, by now, than to speak of the impact of the bone structure on the human body.”[1] For more than a century, policymakers have encouraged, facilitated, regulated, and taxed the production and use of automobiles.
But now, policies unprecedented in scope and consequence are planned to ban the sale of the type of vehicle that 99% of people use—that is, vehicles powered by an internal combustion engine (ICE). Instead, government policies are being launched to mandate, directly and indirectly, electric vehicles (EVs).
Rarely has a government, at least the U.S. government, banned specific products or behaviors that are so widely used or undertaken. Indeed, there have been only two comparably far-reaching bans in U.S. history: the Eighteenth Amendment to the U.S. Constitution, which prohibited the consumption of alcohol (repealed by the Twenty-First Amendment); and the 1974 law prohibiting driving faster than 55 mph. Neither achieved its goals; both were widely flouted, and the first one engendered unintended consequences, not least of which was criminal behavior.
The idea of banning the internal combustion engine (ICE)—or the de facto equivalent through Environmental Protection Agency (EPA) rules—emerges from the thesis that an “energy transition” eliminating hydrocarbon use is both necessary and inevitable. The ICE ban echoes other energy transition ideas but with an important distinction. Electricity production mandates, for example, that ban the use of coal and even natural gas “merely” raise costs, while the product that consumers use, kilowatt-hours, remains largely unchanged in its utility.[2] EVs, as we will explain, do not have the same utility and are neither operationally nor economically equal for most citizens. Nonetheless, hundreds of billions of dollars in taxpayer funds and corporate spending are now being directed at subsidizing and building EVs in concert with many near-term prohibitions on the sale of ICE vehicles.
Enthusiasts rightly credit Elon Musk with launching today’s excitement about EVs. Until the 2012 introduction of the Tesla S—coincidentally, exactly 100 years after Studebaker closed its EV production line, then the biggest EV producer—no company had successfully introduced a battery-only option for an on-road car. Nor, in a century, has any new car company succeeded in taking market share from the legacy competition. Last year, Tesla was the number-one luxury brand in the U.S., accounting for nearly one-fifth of sales in that coveted, profitable category. This was a laudable feat, even if that category accounts for less than 10% of overall car sales.[3] Unsurprisingly, every luxury automaker has scrambled to offer an all-electric option.
We even see nonautomotive tech companies eager to join the fray in building luxury electric cars. Rumors continue that Apple will yet unveil an EV.[4] (Indeed, the company could leapfrog the manufacturing challenge by using 10% of its cash to buy an entire company such as Hyundai.) Xiaomi, China’s “Apple” and the world’s number-three smartphone maker, announced a $10 billion plan to form an EV subsidiary.[5] Meanwhile, conventional automakers have already brought to market more than 40 different EV models.[6]
The arrival of useful EVs didn’t happen because of government mandates or incentives. It was made possible by the maturation of two enabling technologies that were invented in the mid-1970s. One of them, the now-famous lithium battery chemistry, was first identified by Stanley Whittingham, while he was working at Exxon’s New Jersey research labs. (Whittingham was one of three to later receive the 2019 Nobel Prize in chemistry.) The other, less well-known but pivotal (and contemporaneous), invention came from Jay Baliga, who, while working at GE’s R&D center, invented the IGBT, a new class of silicon transistor capable of managing high-power electrical flows. The IGBT made possible the compact, efficient digital control of electrical power critical for all EV drivetrains. Baliga received the 2015 Global Energy Prize for “one of the most important innovations for the control and distribution of energy.”[7]
Useful EVs are undeniably a significant addition to the pantheon of options for consumers. But the rhetoric and policies about the inevitability of EVs for everyone emerge from myths, misperceptions, and hyperbole about the underlying technologies. The International Energy Agency (IEA), for example, begins its 2023 “Global EV Outlook” by touting that EV “markets are seeing exponential growth as sales exceeded 10 million in 2022”[8] (emphasis added). The finger-on-the-scale and hyperbole surrounding EVs begin right there.
Hybrids, as IEA observes in a footnote, accounted for nearly one-third of those global EV sales. Hybrids, by definition, use combustion engines that policymakers are eager to ban. And, relevant to the IEA claim that EV sales have “profound implications”[9] for climate goals, nearly two-thirds of global sales were in China, which is, a priori, a special case—not least because its current and planned coal-dominated electric grid has profoundly negative implications in neutering climate goals.
Nonetheless, the 7 million (non-hybrid) EVs sold globally last year did constitute a huge jump from the 3,000 units sold by Tesla in 2012. And, while only 10% of all EV sales were in the United States (two-thirds of which were Teslas),[10] policymakers appear confident that the “exponential” growth of EVs will make bans and mandates politically palatable because of the EV’s ostensibly inevitable superiority.[11] Aside from the claim of clear superiority—an issue that is a key focus of this report—we note that rhetoric about growth in EV sales being remarkable or “exponential” itself isn’t supported by the history of consumers embracing other new category-creating cars.
It took six years after its introduction before Tesla sold its 200,000th car. Two years after Ford introduced its electric Mustang Mach-E, sales reached only 150,000 (now the distant second most popular EV in America).[12] Compare that to 1983, when Chrysler invented the minivan, well-timed to meet a demographic shift; consumers bought more than 200,000 in one year. But the consumer adoption record belongs to the 1964 Mustang, another category-creating car and one well-timed to meet the demographic shift of that era. Ford sold 1 million Mustangs within 18 months.[13] It took Tesla 92 months to reach that number.[14]
This report does not focus on whether EVs are a practical and appealing new category for many drivers. They are. The world will see tens of millions more EVs on roads even without government mandates. But in banning ICE cars and mandating the use of EVs, policymakers are explicitly betting on the truth of three crucial claims:
- EVs will lead to “profound” reductions in CO2 emissions
- EVs are now, or will soon be, cheaper than, and operationally equal to, ICE cars
- There is a diminishing role for the automobile in modern times; in effect, there is a generational realignment in how citizens seek personal mobility.
All three are bad bets not supported by facts. Before turning to the realities of EV emissions, it is useful to consider the state of mobility as seen in U.S. driving trends. How much, where, and why people drive reveals the kinds of features actually sought in cars.
The Current State and Future of Personal Mobility
ICE prohibitionists are the same as, or at least intellectual fellow travelers with, those who claim that we’ve reached “peak car.” The argument here is that millennials (born 1981–96) and Gen Zs (born 1997–2012) don’t share the affection for cars of baby boomers (born 1946–64). The former two cohorts are ostensibly eager to embrace ride-sharing, bicycles, scooters, and mass transit. Headlines have touted that the “Western world has turned its back on car culture.”[15] Goldman Sachs analysts write: “Millennials have been reluctant to buy items such as cars” and are “turning to a new set of services that provide access to products without the burdens of ownership, giving rise to what’s being called a ‘sharing economy.’”[16] Pundits, especially post-Covid lockdown, intone that remote work will reduce the number of trips that people will take.[17]
The data show that there is nothing to the belief that people in general, or in the rising generation, are giving up driving. Millennials—the first generation of the Internet era—now constitute the largest share of the population. It is thus notable, according to a recent MIT analysis, that, compared with boomers, millennials exhibit “little difference in preferences for vehicle ownership” and that “in contrast to anecdotes, we find higher usage in terms of vehicle miles traveled.”[18] The share of cars bought by the yet-to-come-of-age Gen Zs has increased fivefold in the past five years.[19] The data also show that once the 2008 recession ended and millennials found work, they bought cars and took to the roads along with everyone else, restoring and even somewhat accelerating the long-run growth in total vehicle miles driven on America’s roads. Only the Great Recession, and then the draconian pandemic lockdown measures, put temporary halts on that trend (Figure 1).
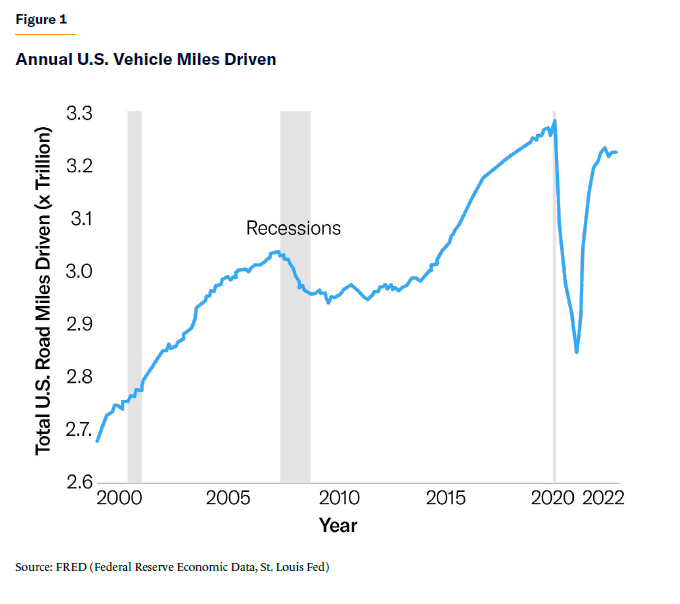
There is also a theory that the Internet causes a decline in car usage. Yet in 1999—at the first peak of digital enthusiasm—British sociologist John Urry presciently claimed that “travel through one medium overall increases travel through other media.”[20] Looking at the long history of “automobility,” Urry wrote that “most car journeys now made were never made by public transport. Car drivers undertake connections with other peoples and places that were not undertaken previously.” And that’s what the record shows: both more driving and more digital traffic.
Another pillar of the peak-car thesis is that urbanization diminishes the need for cars, especially the need for people to drive long distances. Census data, however, show that the urbanization trend ended around 2010, when net migration to nonmetro and rural areas began.[21] While that trend was briefly accelerated by the lockdowns, the net migration to rural and ex-urban zip codes reverted to the trend “observed prior to the pandemic.”[22] As one researcher noted in 2022, the de-urbanization trend could “become more commonplace” if late millennials and Gen Zs follow evidence suggesting that a rising share find “suburban and small-town life more attractive”[23] (Figure 2).
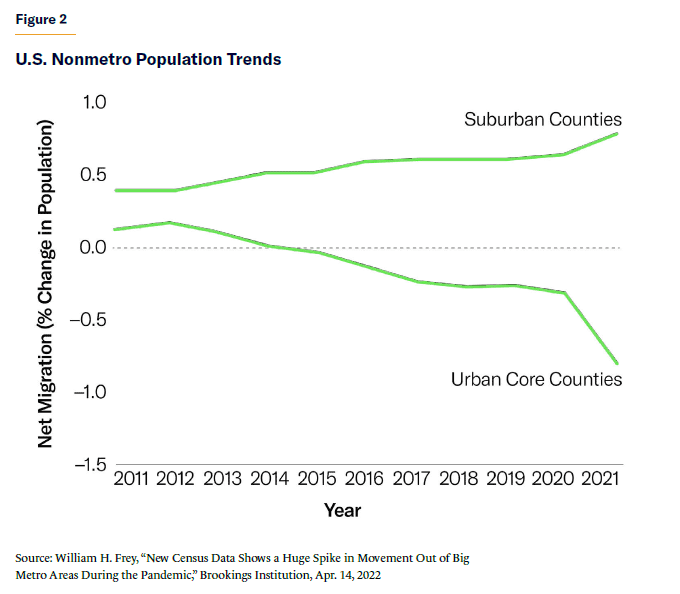
Surveys reveal that not everyone who left the cities during the pandemic will move back, so the trend toward slow de-urbanization appears sticky. Other surveys also show that 60% of Americans claim that they prefer living in suburbs or rural areas and that, even if “money were no object,” only 40% would choose city life.[24] A survey in early 2023 found that two-thirds of Americans would “consider moving to a rural home or a subdivision” if telecommuting were an option.[25] Modern “zooming” has amplified Urry’s synergy of travel in the two different “media.”
One directly measurable effect of these trends is the boom in “super-commuters,” the nearly 5 million Americans now commuting some 90 minutes or longer.[26] Over the last decade, the super-commuting share of the workforce has increased threefold faster than the overall workforce. A 2023 survey by Upwork found that 41% of people are planning to move between two and four hours away from their current residences.[27] Whether telecommuting while living and driving in spread-out ex-urban areas, or super-commuting to urban areas, there is a significant increase in the population living where distances traveled are radically greater than in the city.
Consumer preferences for comfort, size, convenience, and performance have driven a remarkable transformation in the average car purchased in America. Marked from 1975—the dawn of the heavy federal hand in vehicle energy-related regulations—the average automobile today has 100 more horsepower, weighs 1,000 pounds more, and has doubled in fuel efficiency.[28] That last factor means that average CO2 emissions per mile have dropped by half.
Today, for the average household, personal mobility is the number-two expense after mortgage or rent. A car is the single most expensive product that 98% of consumers ever purchase.[29] Banning ICE vehicles would constitute a takeover of one of the top three economic sectors of the nation, bigger than commercial banking or pharmaceuticals.[30]
Now, in service of government climate strategies to achieve radical emissions reductions, consumers will need to adopt EVs at a scale and velocity 10 times greater and faster than the introduction of any new model of car in history. Policymakers are right about at least one thing: that won’t happen naturally from market forces or consumer preferences.
EV Emissions: Elsewhere, Unclear, and Maybe Unknowable
In contrast to cars with internal combustion engines, it’s impossible to measure an EV’s CO2 emissions. While, self-evidently, there are no emissions while driving an EV, emissions occur elsewhere—before the first mile is ever driven and when the vehicle is parked to refuel.
The CO2 emissions directly associated with EVs begin with all the upstream industrial processes needed to acquire materials and fabricate the battery. The received wisdom that EVs will have a “huge impact” on reducing emissions is, whether the claimants know it or not, anchored in assumptions about the quantities and varieties of materials mined, processed, and refined to make the battery.
The scale of those upstream emissions emerges from the fact that a typical EV battery weighs about 1,000 pounds and replaces a fuel tank holding about 80 pounds of gasoline.[31] That half-ton battery is made from a wide range of minerals, including copper, nickel, aluminum, graphite, cobalt, manganese, and, of course, lithium. Critically, the combined quantity of these specialty and so-called energy minerals is 10-fold greater in building an EV, compared with an ICE car.[32]
As researchers at the U.S. Argonne National Labs have pointed out, the relevant emissions data on such materials “remain meager to nonexistent, forcing researchers to resort to engineering calculations or approximations.”[33] And, per IEA, data on the emissions intensity of specific minerals can “vary considerably across companies and regions.”[34] That is a consequential understatement. The fundamental fact to keep in mind: every claim for EVs reducing emissions is a rough estimate or an outright guess based on averages, approximations, or aspirations. The estimates entail myriad known unknowns about what happens upstream to obtain and process materials to fabricate the giant battery. Those factors not only vary wildly but can be big enough, alone, to wipe out from one-half to all the emissions saved by not burning gasoline.
These features of EV emissions constitute a complete inversion of the locus and, critically, the transparency and certainty compared with combustion vehicles. For a conventional car, you know the emissions if you know the fuel mileage. The quantity of gasoline burned is directly measurable and forecastable with precision. Those CO2 emissions are the same regardless of when or where a car is refueled, or when it is driven.[35] And while conventional cars also have “hidden” upstream emissions—the energy used to build the vehicle and create gasoline—these constitute only 10%–20% of these vehicles’ total life-cycle emissions.
The critical factor for estimating upstream EV emissions starts with knowing the energy used to access and fabricate battery materials, all of which are more energy-intensive (and more expensive) than the iron and steel that make up 85% of the weight of a conventional vehicle.[36] The energy used to produce a pound of copper, nickel, and aluminum, for example, is two to three times greater than steel.[37] Estimates of the aggregate energy cost to fabricate an EV battery vary threefold but, for context, on average, the energy equivalent of about 300 gallons of oil is used to fabricate a quantity of batteries capable of storing the energy contained in a single gallon of gasoline.[38]
That so much upstream energy is necessarily used is understandable if one knows that hundreds of thousands of pounds of rock and materials are mined, moved, and processed to create the intermediate and final refined minerals to fabricate a single thousand-pound battery (see sidebar, “Sources of ‘Hidden’ Energy to Mine and Process 500,000 Pounds per EV Battery”).
Accurately estimating the actual quantities of specific fuels used is complicated by the labyrinth of global suppliers and the lack of transparency with many of the companies. (There is far greater transparency and accuracy in tracking embodied energy for iron and steel, since at least three-fourths of production is domestic.)[39] Without knowing all that, no one knows the ultimate real-world emissions from making an EV. While dozens of technical papers have been devoted to analyzing uncertainties around these upstream emissions, a useful starting point is IEA’s seminal report on “energy minerals,” which includes both upstream factors and grid fueling. The agency concludes that, compared with an ICE car, there is still about a 50% reduction in life-cycle emissions for EVs[40] (Figure 3).
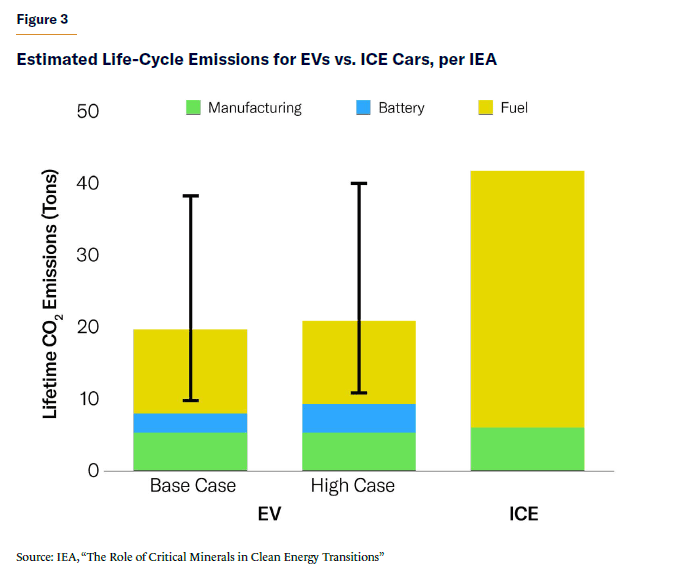
However, the IEA analysis not only uses debatable assumptions but also buries the variables and uncertainties. While IEA does illustrate variables (as black vertical “error bars” in Figure 3, or as a range of outcomes in another analysis)[41] the agency actively promotes the claim—indeed, essentially a guarantee of “profound” emissions reductions—even though its own estimates show that an EV could yield no reductions at all, or even an increase. As a practical matter, there is no way to know where on that range any vehicle, or even most vehicles, would perform. The appearance of certainty or precision—an average 50% reduction—is illusory.
One dubious factor in the IEA estimate is the assumption about the battery size; its calculation is based on a 40 kWh (kilowatt-hour) battery pack, which is half the size of the batteries in most popular EVs.[42] In fact, IEA itself notes elsewhere that SUVs with big batteries account for 60% of all EV options (excluding China, where it’s 40%).[43] Bigger batteries for more range mean that more materials are used and thus entail greater upstream emissions. The IEA calculation also ignores the greater use of aluminum for an EV body and frame (done to minimize the overall weight penalty imposed by the battery), which also adds to the upstream emissions because manufacturing that metal is so energy-intensive.
Consider, instead, estimates offered by Volvo and an EU-funded analysis of Volkswagen’s e-Golf, the latter a small sedan using a small battery.[44] The e-Golf’s upstream emissions, combined with emissions from the power plants that supply EU electricity, yield cumulative CO2 emissions greater than the diesel version of that car for the first 60,000 miles of driving. After 120,000 miles, the accumulated emissions for the EV are estimated to be about 20% lower than the ICE version (Figure 4).[45]
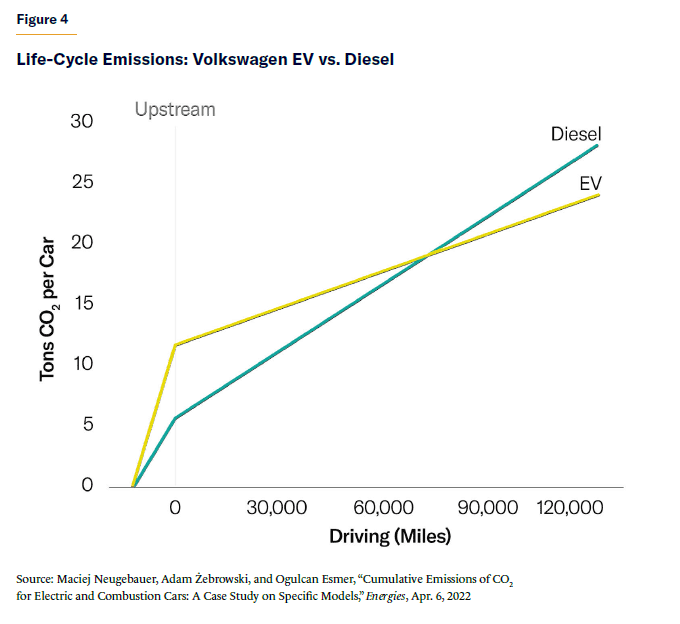
Volvo’s study compares that company’s Recharge SUV using a 69 kWh midsize battery pack with its not particularly efficient gasoline-driven XC40 SUV.[46] The Volvo’s upstream emissions estimate is double that of the VW e-Golf, mainly because of a bigger battery. And similar to the VW analysis, Volvo found that its EV had greater total emissions than the comparison gasoline vehicle for the first 45,000 miles of driving (again, including the average emissions from electricity supplied on the EU grid). After 120,000 miles, Volvo estimates that its EV has a cumulative emissions reduction of about 30% (Figure 5).
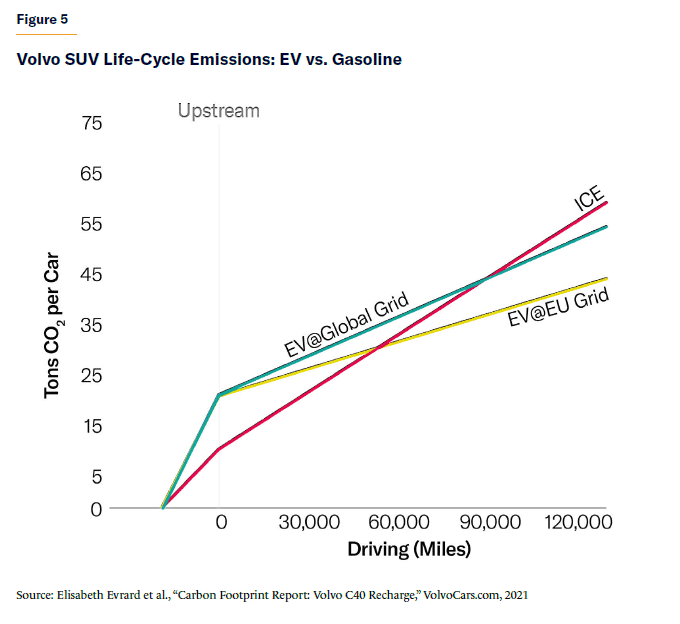
Such estimated life-cycle emissions savings can shrink or evaporate if one assumes an EV using the more common, bigger battery sizes that consumers prefer. The “choice of methodology,” as Volvo’s study noted, “has a significant impact on the total carbon footprint.”[47] Volvo, for example, assumed a “global average electricity mix” for emissions arising from producing and refining battery materials.[48] That may sound reasonable but doesn’t reflect the actual emissions where most of those materials are processed, which, given supply-chain realities, are often on coal-dominated grids.
In another example of illusory precision, the Wall Street Journal—in a 2021 investigation seeking to unearth the truth about EV emissions—asserted that even accounting for grids and materials, “the data show that switching from gas to electric vehicles will make a huge impact.”[49] But that confidence was based on a study that the newspaper commissioned from a University of Toronto team.[50] For the value of the upstream emissions from fabricating a battery, that study used a number at the lowest end of the known range for such emissions, the upper end of which is 250% greater.
The WSJ was in good company with its confident conclusion, since IEA also asserts that emissions “along the mineral supply chain do not negate the clear climate advantages of clean energy technologies,”[51] a claim parroted by governments and proponents and enshrined in mandates and bans. The fact is—as the data show, including in IEA’s reports—it’s anything but clear.
Upstream Emissions: Known Unknowns
A technical review of 50 different analyses reveals that the bottom lines for embodied EV emissions vary by a factor of five.[52] It’s meaningless to use an average number for such a wide range. Actual EV life-cycle emissions are dominated by the assumptions made for three key variables:
- The size of the battery pack
The 50-study review found that a 30 kWh battery pack was the median size analyzed and that life-cycle emissions were “rarely” calculated for large vehicles and batteries that consumers commonly choose.[53] In 2022, Teslas and the Mustang Mach-E accounted for nearly three-fourths of EVs bought by U.S. consumers and have 60–90 kWh batteries.[54]
- The location of the mines
The energy used to obtain a pound of metal depends on the mineral ore grades, the size and nature of a mine, the distances that materials are transported, and the nature of the grids and fuels used at specific mines. For copper, that number can vary at least twofold and for nickel by threefold.[55] Getting accurate information is complicated by the fact that 80%–90% of relevant minerals are mined outside the U.S. and EU.
- The location of the refineries
The energy/emissions for specific mineral refineries vary with their age and the design of the refineries, as well as the location—and thus grids—used. Some analyses of life-cycle emissions make implausible assumptions—such as emissions from a hypothetical “average global grid”—or, for example, that the aluminum used to build U.S. EVs would come from North American production on grids that are 80% hydroelectric.[56] In reality, China refines 50%–90% of the world’s suite of energy minerals.[57] The CO2 emissions associated with refineries in China are 1.5x greater than those in the EU or United States.[58]
EV proponents imagine adding clarity to the materials sourcing issues by creating rules and regulations and offering sensors and software that could, in theory, document the relevant data. Such prospects are, at best, remote, given the political, economic, and privacy challenges of such a global pursuit. Transparency in energy minerals—never mind an imposition of “clean” practices—is a far more daunting task than, say, ensuring that imported diamonds are “conflict-free.”
Keep in mind that all these uncertainties are baked in before making guesses about variables in where and when an EV is fueled. Nonetheless, including the range of known supply-chain possibilities yields possible scenarios wherein an EV driven on grids that will exist for the next decade leads to greater lifetime emissions than using an ICE car (Figure 6).
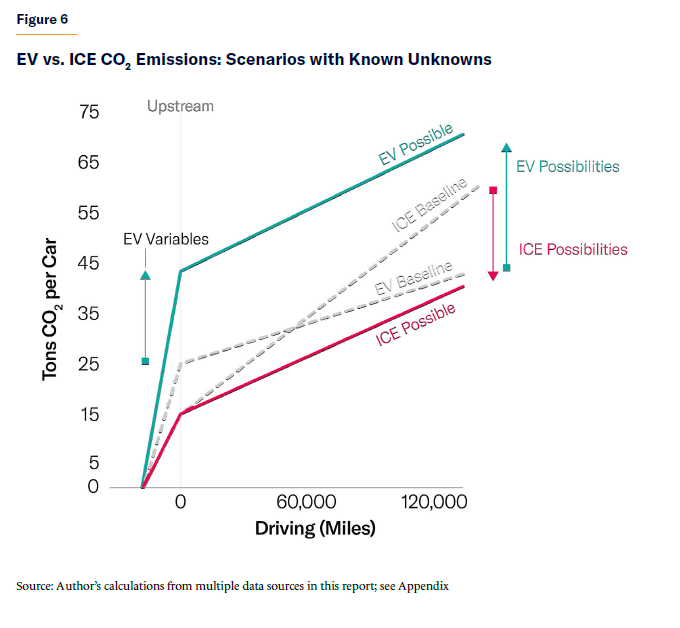
Then there are other variables that, though each individually is a relatively small share of the total picture, are nonetheless collectively important in the calculus of EV emissions. These include:
- Location of the battery and EV assembly factory
A battery plant in Norway, where dams provide about 90% of electricity, adds very little to upstream emissions from mineral processing and assembly, while a lot is added for the same plant in China, where coal supplies two-thirds of grid power. Notably, half the world’s EVs in 2022 were built in China, and China is rapidly entering global markets, selling EVs across the world.[59] So far, there are no plans to repeal the Trump-era 27.5% tariff on Chinese EVs, but Chinese companies like BYD are increasingly exporting low-price (subsidized) vehicles around the world.[60]
- Battery chemistry
There are about a dozen variations in lithium chemistry. While these entail different ratios and types of some minerals, the overall quantity of materials, and thus mining, needed per battery remains roughly the same. The exception is with lower energy-density chemistries. For example, the lithium-iron-phosphate (LPO) chemistry, popular in China and with some automakers because it doesn’t use cobalt or nickel, has a 20% lower energy density. That translates into either a 20% lower driving range or building a bigger, heavier battery requiring more copper, aluminum, polymers, and lithium.
- Materials for the rest of the vehicle
Many analyses assume that the body and frame of the EV have the same embodied energy as a conventional car.[61] But the EV’s electric motor and high-power wiring necessarily use several hundred percent more copper than vehicles with iron-and-steel engines and drivetrains. In addition, automakers use more aluminum for the EV frame and body to offset the battery’s weight penalty. Embodied emissions from 300 to 500 extra pounds of aluminum are roughly equal to the emissions from driving 10,000 miles in a conventional car.[62]
- Emissions from EV power electronics
An EV uses about 200% more electronics for power management. Silicon device fabrication is extremely energy-intensive (about 100x more, pound-for-pound, than steel), but, as one analysis put it, energy-use “data for electronics production still needs to become better.”[63] The data available suggest that the uncounted CO2 emissions embodied in each EV’s power electronics roughly equal those from driving an ICE car 3,000 miles.[64]
- Battery life span
Most analysts assume that a battery pack will last the EV’s lifetime, but life spans depend on how consumers charge the battery—fast or overnight. As one study put it, using “intensive,” i.e., on-road fast charging, rather than “light” overnight charging, typically cuts a battery’s life in half. Modeling the emissions from an EV fleet requires estimating what share of owners will need two batteries per car life span.[65]
- Total miles
When ICE cars and EV emissions are expressed in mileage terms, i.e., grams of CO2 per mile, that calculation necessarily assumes a number for total lifetime miles. Spreading out the upstream emissions over more lifetime miles arithmetically improves the CO2 mileage. (An ICE-car CO2 mileage is invariant with total miles assumed.) So far, data show that EVs are driven half as many miles per year as ICE cars. Using that lower mileage arithmetically doubles the EV’s rated emissions per mile.[66]
- ICE fuel efficiency
When EV emissions are presented as a percentage reduction over an ICE car, one assumes a fuel mileage for the latter. But realistic forecasts would incorporate future trends in combustion engine efficiency. An analysis of engine technology progress finds 30%–50% fuel efficiency gains will be on offer by 2030 and thus an equal reduction in CO2 emissions per mile. Using the performance of a future ICE for comparison with a future EV further closes any gap in estimated emissions savings (Figure 7).
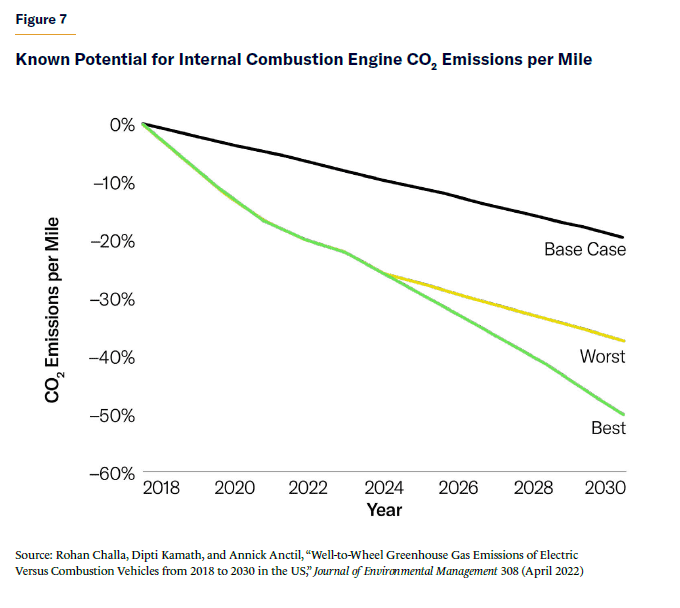
Returning to the upstream materials, the dominant variable affecting EV emissions: everything about the future of EV emissions is anchored in mining, humanity’s oldest industry. Thus, by definition, estimating future EV emissions requires guesses about the future of global mining, where we find yet another array of known unknowns.
Future EV Upstream Emissions Are All About Mining, Not Recycling
Because of the astonishing magnitude of minerals needed to build an all-EV future, estimating emissions will be completely dominated by assumptions about the locations and nature of new mines and refineries. For context: the materials needed to build EVs for the world’s 70 million cars sold per year equals about 500 years of the materials now used to fabricate the 1 billion smartphone batteries now produced annually.
Hundreds of megatons of earth will need to be dug up and processed in the service of EV goals.[67] To meet “transition goals,” the materials demanded will require supplies to rise 400%–4,000%, depending on the mineral. IEA says that will require hundreds of new mines. Benchmark Mineral Intelligence puts the number at 384 new mines just to meet EV needs for the “graphite, lithium, nickel, and cobalt [that] will be required to meet EV demand by 2035.”[68] (Similar expansion will be needed for similar minerals to build solar panels and wind turbines.) EV proponents commonly invoke recycling as the solution to these monumental materials and emissions impacts, especially the holy grail of a “circular economy,” i.e., 100% recycling.
Recycling will be irrelevant for a long time, as far as mitigating upstream minerals demands. Since manufacturers claim that EV batteries will last a decade, that means that there won’t be much of anything available to begin recycling until the early 2030s.[69] The best that IEA could come up with is recycled minerals meeting 1%–2% of battery demand by 2030.[70] As for the following decades, enthusiasts’ unrealistic dream of perfect recycling, even were it feasible, would still leave the need for an astronomical rise in overall minerals supplied.[71]
The central and largely ignored issue for upstream emissions accounting is not just the known fact that minerals demand will rise but the other known fact that for future mines and refineries, emissions are increasing per pound of mineral.[72]
Meanwhile, Upstream EV Emissions Are Rising
For all of history, the costs of a metal in both dollar and environmental terms are dictated primarily by ore grades, i.e., the share of the rock dug up that contains the metal sought. (Also related is the depth of the ore and thus the quantity of “overburden”—the rocks, dirt, trees, etc., on top of the ore—that must first be removed.) Ore grade is what accounts for the differences in the cost per pound of gold, $15,000, and iron, $0.05. The former ore grades are typically below 0.001% and the latter over 50%.[73]
Iron (and aluminum) are uniquely abundant metals; not so the suite of critical “energy minerals,” for which ore grades range from 2% to 0.1%. Average nickel ore grade is under 2% and for copper below 1%, which means, arithmetically, that at least one ton of rock (excluding the overburden) must be dug up, ground up, and processed to obtain, respectively, 40 pounds and 20 pounds of metal.[74] Such geological realities determine the amount of energy used by big machines to do the digging, moving, grinding, refining, etc.
The global mining industry today already accounts for about 40% of all industrial energy use, and that’s before an epic expansion that will be needed to meet green plans.[75] Petroleum itself typically accounts for half of mining-sector energy use.[76]
Thus, estimating future EV energy emissions requires including the trajectory for ore grades. There is no evidence that any study is doing so.
Every metal exhibits a long-run and significant decline in ore grades. IEA acknowledges this, even if it tendentiously understates the reality: “Future [minerals] production is likely to gravitate towards more energy-intensive pathways.”[77] The word “likely” dodges the fact that the data and the trends are clear. Copper is typical and is one metal for which there are no substitutes for building EVs or wind and solar hardware. As a National Renewable Energy Laboratory paper pointed out, “a decrease in copper ore grade between 0.2% and 0.4%, will require seven times more energy than present-day operations.”[78] And copper ore grades are forecast to continue the long-run decline (Figure 8).
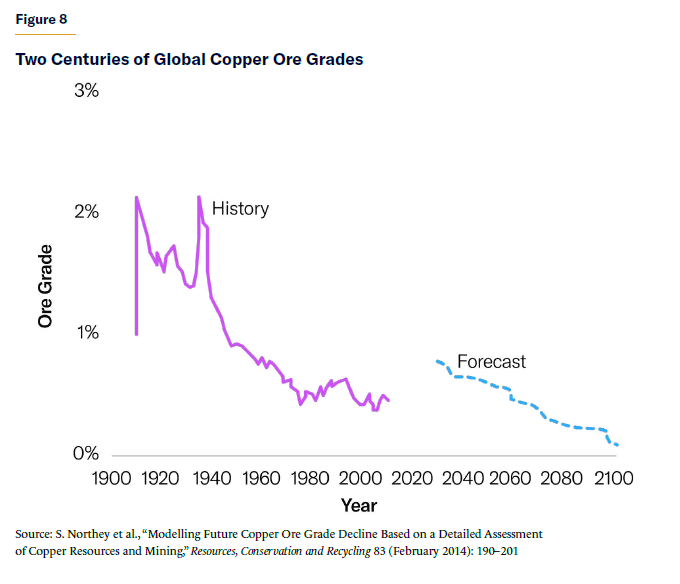
A World Bank study of EV upstream realities acknowledged the flaws in any emissions prediction. Even its own analysis, it notes, was based on “historical sources and are static” and didn’t “take into account . . . declining ore grades.” The bank called for “future research.”[79] But much of that research is available now. For example, according to IEA’s “Role of Critical Minerals” report:
- “Lithium production has been moving from brine-based recovery (mostly in Chile) to mineral concentrate production from hardrock (mostly in Australia). The emissions intensity of hardrock-based lithium carbonate production is three times higher than that of brine production.”
- “Demand is moving from lithium carbonate towards lithium hydroxide, as the latter is more suitable for batteries with higher nickel cathode chemistries. However, lithium hydroxide involves more emissions.” Notably, the nickel-based chemistry is pursued to avoid cobalt because of human rights concerns within the Congo, the dominant global cobalt source.
- “Battery-grade nickel faces a similar situation. While sulfide resources played a major role in the past, future growth is increasingly coming from laterite resources, which require more energy to produce.”[80]
All the trends for declining ore grades are visible, even if they are ignored. Consider copper again and the trends in Chile, the world’s biggest supplier, where average ore grade decreased by about 25% over the decade 2003–2013.[81] In those years, total copper-associated energy use—and thus emissions—grew twice as much as the growth in tons of copper supplied (Figure 9).
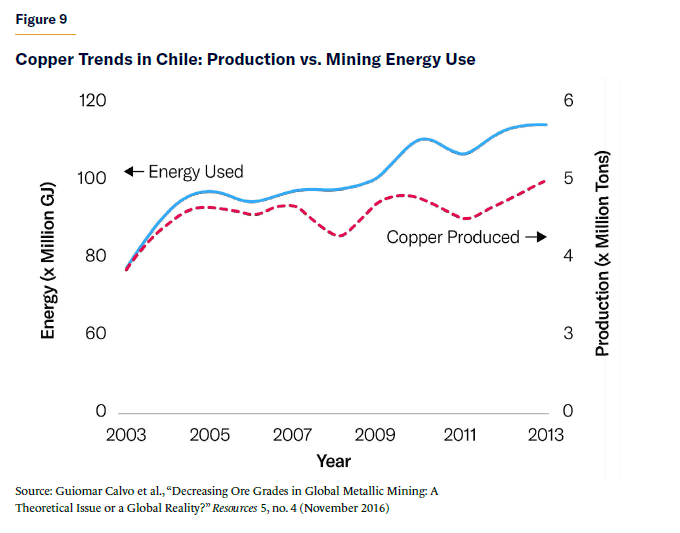
There’s No Magic for Fixing the Battery’s Carbon Debt
Technology offers ways to mitigate rising upstream emissions: better battery chemistry to reduce the materials used to store energy, more efficient refining processes, electrified mining trucks, and wind and solar electricity at mine sites. But none of these factors can have a significant impact in the time frames contemplated for the rapidly expanding EV production demanded by current transition mandates.
News stories serially claim a “breakthrough” in battery technology, but there are no commercially viable alternative battery chemistries that significantly change the magnitude of the physical materials needed. To meaningfully reduce primary mineral demands would require a nearly 10-fold leap in underlying electrochemistry efficiency. Such gains aren’t even theoretically feasible.[82] Still, it is reasonable to imagine that some foundationally superior innovation will emerge; even so, many years would be needed to safely scale up industrial chemical systems. It was three decades from the discovery of a lithium battery to the first Tesla sedan. Batteries used in the near future will necessarily use technologies available now.
Similar realities constrain the prospects for radical efficiency gains in the various industrial mineral-refining processes. While incremental improvements are inevitable, especially in the digital era, there are no known “step function” gains visible in these well-trod fields of physical chemistry. Many processes are already operating near physics limits.[83] As a portent of the future, energy savings in the modern history of mining and mineral process efficiency have been wiped out by declining ore grades requiring larger volumes of ore needed to yield the same pound of metal (Figure 10).
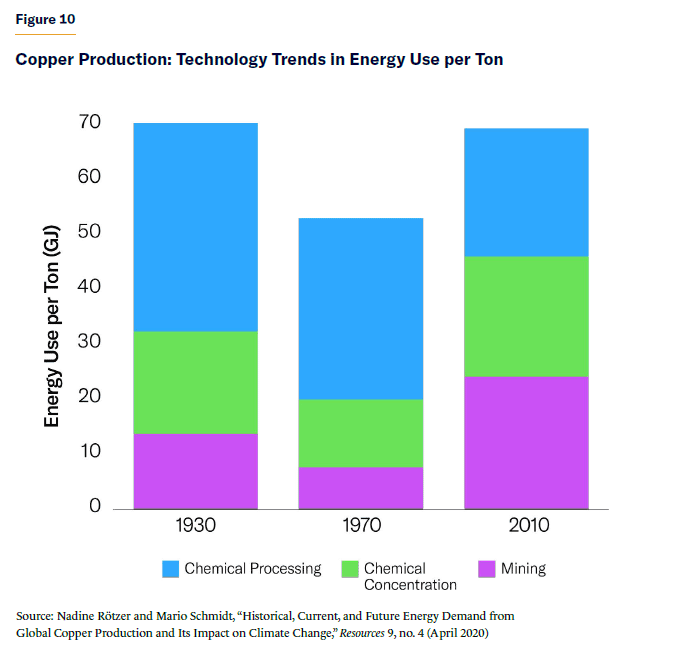
Caterpillar, Deere, and Case (and others) all have projects for electrifying mining trucks. Promising designs are on the horizon for specific applications, but batteries for most heavy equipment are not up to 24x7 performance and industrial-class demands—never mind costs.[84] And the turnover rate for mining and industrial equipment is measured in decades. Mines will use a lot of combustion-centric equipment for a very long time.
Meanwhile, pressures to rapidly increase mineral supplies will push developers to build smaller mines that are inherently faster and cheaper to build.[85] But smaller mines typically entail greater use of trucks; only big mines can justify the infrastructure costs of building dedicated (energy-efficient) rail systems. And as IEA has also pointed out, over half the electricity used in industry is not grid-connected but produced on-site, and much of it with diesel-fueled generators, especially at remote and small mines. Such on-site generation accounts for two-thirds of mining electricity in China and 95% in the rest of Asia.[86] While some mines are installing wind and solar systems, the inherently higher costs of these power sources will impose limits because energy spending accounts for about 40% of overall mining costs, setting aside the practicalities of 24x7 power needed at mine sites.[87]
Over the longer run, a foundational transformation in industrial technology efficiency is coming, one that echoes that of a century ago. This time, the source will be Cloud-centric, networked machines mediated by artificial intelligence, along with new classes of autonomous smart trucks and robots.[88] But those advances won’t significantly change the realities in time to meet the hypertrophied demands for industrial minerals within this decade.
The trends that are knowable for the near future show upstream emissions rising for EVs, even if we don’t know exactly how much they will increase. Gaining clarity will be a challenge not least because, as IEA has noted, only a tiny share of players in that industry are cooperating with “emission pledges” made for the Responsible Mining Index.[89] The solution that IEA offers is “greater pressure from governments, investors, and end-use customers.” We doubt that leads to clarity anytime soon.
Meanwhile, the upstream emissions uncertainties are multiplied by yet more variables: how EVs will be fueled at scale.
Kilowatt-Hours and Elsewhere Emissions: More Known Unknowns
Emissions from refueling an EV vary wildly, depending on the where and when, thanks to differences among grids, combined with hourly variations in electricity production. Accounting for those realities, and not a hypothetical average kWh, reveals that CO2 emissions per EV fill-up can range from zero to as high as the same as just burning gasoline to drive the same number of miles.
Current estimates of EV on-road emissions are nearly universally anchored in a simple calculation based on the average emissions from a kWh produced on a local grid, multiplied by a vehicle’s rated kWh mileage. As with upstream emissions numbers, refueling numbers also give an illusion of precision.
Because of grid variabilities, the actual (not average) emissions from producing a kWh varies dramatically, depending on the hour a battery is charged, a feature that will be complicated as more wind and solar are used. No such variability comes from ICE cars; calculating CO2 emissions based on gallons consumed is essentially the same whenever gasoline is purchased, produced, or burned.
Similar uncertainties and disparities are associated with the mileage numbers used to calculate emissions. In the real world, an EV’s kWh/mile varies quite significantly from a vehicle’s claimed rating—again, unlike the minimal variability associated with ICE-car fuel mileage.
The uncertainties start with the EPA “sticker” mileage provided for consumers (and estimators). A recent study found the actual on-road EV mileage averaged 12.5% worse than the certified window-sticker rating. For ICE cars, however, the average, real-world mileage per gallon was 4% better than the EPA sticker.[90]
More significantly, EV mileage is about 30% worse when it’s 20oF outside, versus 80oF, because battery electrochemical reactions are unavoidably slow at lower temperatures.[91] There’s only a 5% drop in fuel efficiency for ICE cars over the same temperature range. On top of that, as road testers and consumers know, using an EV’s heater in winter can lower kWh mileage by as much as 50%.[92] (An ICE car scavenges free waste heat.) Such temperature factors are generally ignored, not only on EV “sticker” ratings but especially so in estimating national emissions impacts from EVs. Those factors matter, since one-third of the U.S. population lives in cold northern latitudes.[93] Odds are that EPA will eventually sort out accurate ways to rate EV mileage. But unlike conventional cars, the EV sticker might need to be accompanied by a map with a rating, say, for each state where it’s driven, based on the share of the year experiencing low temperatures.
The realities of local grids add another set of uncertainties, something that EPA recognizes on its website with an EV emissions calculator that divides the country into 27 electricity supply regions. There, one finds that the grid assumed leads to a threefold variation in CO2 emissions per kWh of fueling.[94]
Even that EPA calculator understates the magnitude of uncertainties because actual emissions-per-kWh used do not depend on the average for a region but on the specific hour of the day that a battery is charged. The hourly factor is critical with fast chargers that provide a fill-up in under an hour rather than overnight, a feature essential for on-road refueling, in order to allow widespread EV adoption. Hourly emissions per kWh produced can vary by another threefold or more.
Taken all together—time of year and day, and grid specifics—the real-world CO2 emissions per kWh actually used to charge an EV can vary about 10-fold. Emissions from a fill-up could be nearly zero during a sunny summer hour (ignoring the solar electricity’s upstream minerals emissions). Or during an hour when it’s cloudy in the winter, just the fill-up emissions, ignoring upstream factors, could be greater than that from burning gasoline to drive the same distance.[95]
Such disparity exposes the lie of oft-made assertions—for example, in another recent WSJ article: “Regardless of where they are driven, EVs are always a climate-friendlier choice than their gasoline counterparts, according to recent studies published by the European Environment Agency and the International Energy Agency, as well as academic research.”[96] Those studies either ignore or bury in footnotes the reality of variables.
Some EV proponents know better and propose so-called smart-charging to ensure that batteries are charged only when carbon-free electricity is available by using software to track a specific vehicle location and time of charging. That information would then be used to provide incentives to fuel at the “right” time, penalties for refueling at the “wrong” time, or both, or even remotely disabling chargers when consumers are making an unacceptable choice.[97]
Such machinations to influence consumer behavior and freedom are, prima facie, radically different from refueling conventional cars. And such realities illuminate the flaw of claiming that EVs are inevitable because of parity with, if not superiority to, conventional cars.
The Parity Trope: EVs Are Not Yet Equal with ICE Cars, and Won’t Be Anytime Soon
The EV is widely touted as a transition comparable to the shift away from the horse-and-buggy age. But changing how a car is powered has the equivalent relevance to a mobility “revolution” as changing the nature and source of a horse’s feed. And to encourage adoption of the newly invented gasoline-powered cars, governments didn’t have to ban horses.
To be sure, EVs offer features that appeal to millions of drivers, mainly wealthier ones and especially the cohort of car enthusiasts who gush over “insane” acceleration.[98] Equality in all features is neither relevant nor needed for EV sales to grow, as there are many different reasons consumers choose any one of hundreds of different kinds of car models. But the premise of the inevitability of an all-EV future is not just about luxury EVs offering “insane” features, or even about reducing emissions.
The premise is that EVs are superior in every way to the old technology and thus “electric cars are going to take over the world,” as the Wall Street Journal’s automotive columnist, Dan Neil, enthused, or as regulators and policymakers now plan to mandate.[99] The EV is framed not only as leaving the horse era, but also doing so at the same kind of technology acceleration witnessed in computing and communications. Thus, as Neil went on: “Remember flip phones, fax machines and dial-up modems? You want an electric vehicle because they are generationally improved products: quieter, quicker, more refined, more efficient, offering superior vehicle dynamics, less maintenance, and lower per-mile operating costs.” Neil is not alone in invoking the tech analogy. For example, an International Monetary Fund report asserted: “Smartphone substitution seemed no more imminent in the early 2000s than large-scale energy substitution seems today.”[100]
The notion that we’re witnessing a tech-like acceleration for EVs is worse than a canard—it’s nonsensical in the energy physics of moving people and cargo versus moving data. If battery chemistry—the pillar of EV inevitability—could follow the arc of computing’s progress, we would soon see a peanut-size battery power a car for its lifetime on a single charge. Only in comic books does energy tech advance at the pace of information tech, such as in Moore’s Law (Figure 11).
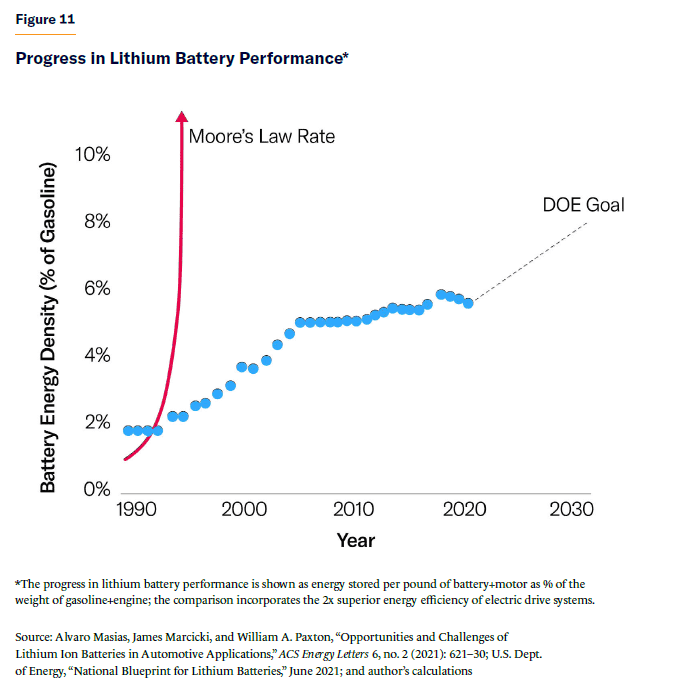
Similarly central to the inevitability and cheaper-better claims is the assertion that EVs are inherently simpler machines and that the “old” ICE technology is maxed out, with no innovation remaining. Again from Mr. Neil: “Compared to EVs’ few moving parts, the complexity of modern gas-powered vehicles terrifies me . . . [and] combustion technology is about as good as it will ever get.”[101]
The reality is otherwise. Yes, conventional cars do have a complex thermo-mechanical system, with the engine and automatic transmission made from hundreds of components, mated with a very simple fuel system, a tank holding a liquid with a one-moving-part pump.[102] EVs, inversely, have a very simple motor made from just a few parts. However, the EV fuel tank is a complex electrochemical system made from hundreds of parts, sometimes thousands, including a cooling system, sensors, and control electronics. In addition, the EV drivetrain requires roughly double the quantity of microcontrollers and power electronics.[103]
As for any claim that we’re at the end-of-innovation for combustion engines, myriad radical advances in ICE technology are already under way, as can be seen in the technical literature as well as from automotive research labs, with many commercially available (if not widely used). Engines with efficiency double that of today’s average have been demonstrated and, in some cases, deployed in nonautomotive applications.[104] Some new engine designs have as few moving parts as an electric motor.[105] Greater advances are still possible with ICE tech than are with electric motors or batteries. In terms of overall mineral resource requirements, a 1% improvement in combustion efficiency equals a 10% advance in battery-electric tech.[106]
It is true enough that the advent of lithium battery chemistry is one of history’s rare technology pivots enabling collateral innovations in many areas, not just cars. But, absent a prohibition of ICE cars, the extent to which EVs capture a majority of the entire automotive market will be determined by three factors:
- Purchase price reaching parity with ICE cars
- Operating costs and convenience reaching parity
- Parity in the macro issues of supply chains and broad environmental impacts.
To understand these factors, consider:
The Future Price of EVs Is in the Hands of (Foreign) Mining & Refining Companies
So far, EVs are sold mainly in the luxury-car category, where sticker prices exceed $60,000.[107] In the U.S., there are no EVs in the lowest vehicle-price category, $16,000–$20,000, where there are 10 models of conventional cars.[108] Nevertheless, the claim that EVs are getting cheaper, fast, and will soon reach parity with (or even be priced lower than) conventional cars is based on the notion that battery prices are on track to “collapse.”[109]
Today, the battery adds at least $10,000 to the price of an EV.[110] Meanwhile, with higher interest rates intersecting rising prices for conventional cars, the Washington Post recently noted that “new cars, once part of the American Dream, [are] now out of reach for many.”[111] Consequently, purchases for “the lowest 20 percent of earners dropped to its lowest level in 11 years. Meanwhile, spending on new cars by the top 20 percent reached its highest level on record.”
The claim of plummeting battery costs is true only in hindsight, measured since the inception of lithium technology; this is a trend typical for all new technologies. But like all technologies in the world of atoms, not bits, after early years on the manufacturing learning curve, the rate of improvement slows dramatically. Over the past half-dozen years, battery cost has decreased slowly; and over the past couple of years, prices have reversed. Battery costs have risen 20% since 2021.[112]
Future battery costs now depend almost entirely on a single fact: basic materials now account for 60%–80% of the cost to make batteries.[113] Even if labor and capital costs decline (both are currently trending up), battery prices (and future upstream emissions) are now firmly in the hands of decisions made by global miners and refiners.
We know that a far greater supply of minerals will be needed to build an all-EV world. As a fusillade of subsidized battery factories come online in the next few years, the demand for (and the cost of extracting) these minerals will skyrocket. In the context of prohibiting ICE cars, the issue is not whether sufficient resources exist on our planet but whether there will be enough mining, soon enough. As IEA reported, it takes 10–16 years to open a new mine.[114] Even the New York Times is now covering the obvious with stories headlined “The U.S. Needs Minerals for Electric Cars. Everyone Else Wants Them Too” and concluding that “the world is facing dramatic long-term shortages by any estimate.”[115]
The data show that the mining industry isn’t remotely close to scaling up the supply needed. Global spending plans to expand or add new mines are roughly 10% of what will be required by 2030 to meet green energy mineral demands[116] (Figure 12).
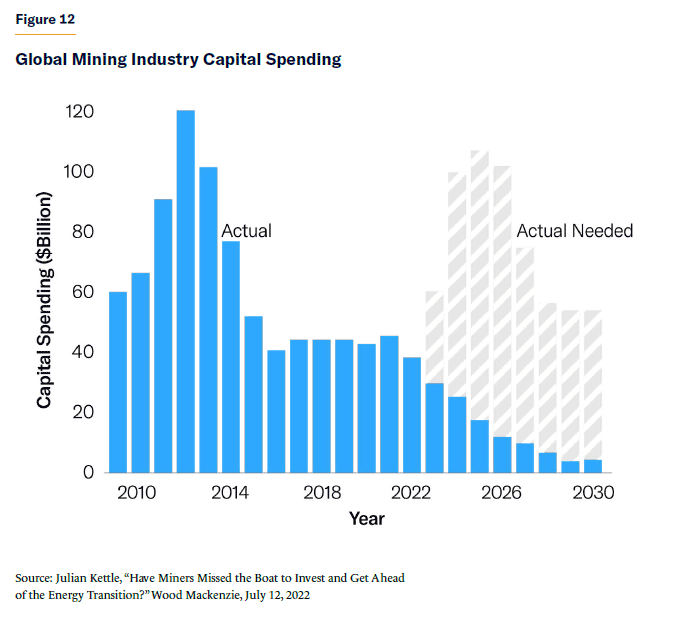
We also know a lot about market concentration. The overwhelming majority of minerals supply is located outside the U.S. and EU.“None of the raw materials required for battery-cell manufacturing are currently mined in significant quantities in Europe,” a recent German government study noted. “Notwithstanding the increase in recycling and European raw material projects that have been announced, this import dependency will remain largely unchanged by 2030.”[117] Precisely the same is true for the United States. Absent radical regulatory reforms (far more than anything enacted or proposed), that reality will remain unchanged regardless of elliptical language in subsidies to encourage reshoring in Europe or America. When it comes to resource supply, some well-known factors are relevant to price trends—factors again rife with unknowns.
For energy minerals, China has double the market share that OPEC has with oil. China is busily expanding mining investments in Africa and South America and is on track to raise its share of the refined lithium market from 24% last year to 32% within two years.[118] Other countries are following Indonesia’s new policy (the world’s top nickel producer), wherein exports of raw mineral ore are prohibited, requiring the construction of local refineries.[119] Meanwhile, in South America, where two-thirds of the world’s low-cost lithium resources are known (and one-third of current production), there’s talk of a Lithium cartel.[120] The effect on prices of such market concentrations are well known. Strategies to form “buyers’ clubs” among nations, recently proposed by the U.S. government in collaboration with the EU, run up against their historical failure. Monopolies, cartels, or dominant sellers invariably have more price control.[121]
Even if the energy-minerals market were to be uniquely free of price manipulation, the basic economics of supply and demand points to dramatic price increases for batteries—as well as other products dependent on these minerals. For many minerals, EV demand is transitioning from a marginal share to the dominant use. Competition for these minerals’ supply, and inevitably price pressures, will begin to have an impact on the cost of building everything from homes and buildings to appliances and computers. Five years ago, EV markets constituted, for example, 15%, 10%, and 2% of all uses for lithium, cobalt, and nickel, respectively; last year, those shares were 60%, 30%, and 10% and rising rapidly.[122]
Using long-run historical and financial data on minerals, IMF economists modeled the price implications from mineral demands entering a period of consistent undersupply due to all the EV and energy transition goals. The study’s unsurprising conclusion: prices for various metals would reach historical peaks “for an unprecedented, sustained period of roughly a decade” (Figure 13).
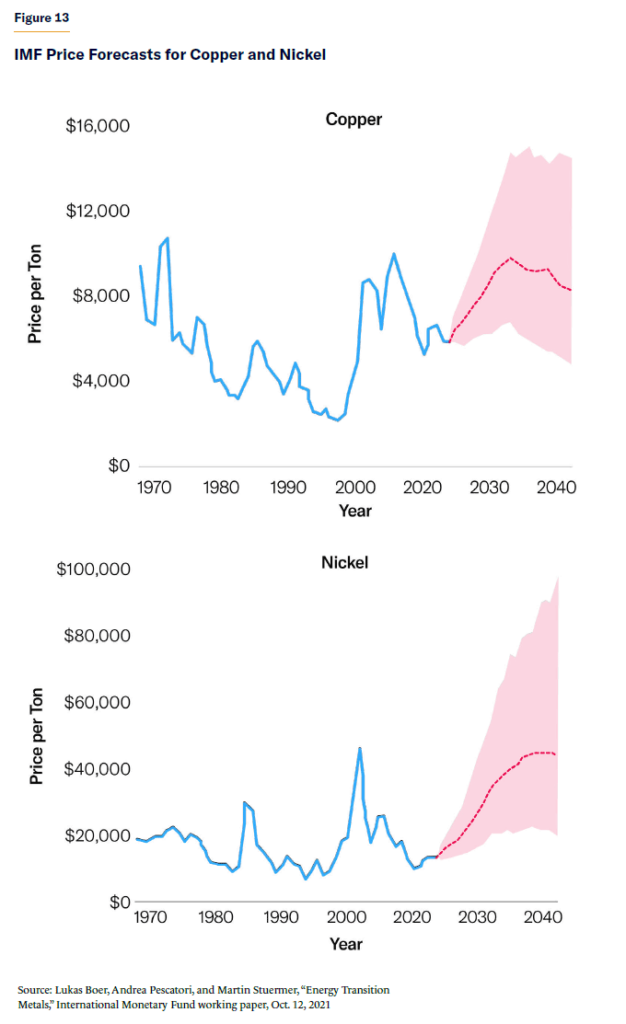
Among the known unknowns, the IMF economists also noted that the “integrated assessment models” for forecasting energy transition economics “do not include the . . . potential rise in [mineral] costs.” Forecasters, as well as policymakers, appear lulled by the 20th century, during which there was a long-run slow decline in the average prices of minerals (net of short-term price volatility). But that trend began to reverse just over a decade ago.[123] Long-term rising mineral prices would be something new not only for EV forecasters but also for economists in general and for the Federal Reserve’s inflation-fighting efforts.[124]
All the underlying minerals trends are hard to square with automakers claiming that they expect to soon see lower production costs for EVs, the key to a transition from money-losing to profitable sales.
Refueling Infrastructures in an All-EV Future
So far, 90% of EVs in the U.S. have been purchased as a second or third car by wealthy households with a garage.[125] A recent JD Powers survey confirms that for luxury-car buyers, “driving performance” was the primary reason for the purchase.[126] For these owners, an exciting-to-drive EV is convenient and inexpensively refueled overnight. Consistent with this market, the data show that most EVs are used only occasionally and driven half as many miles as the average car.[127] For automakers, that’s still a tantalizingly big market because at least 50 million vehicles in the U.S. are put to occasional use by a multicar household.[128] But that’s not the all-EV world that the prohibitions on ICE vehicles intend to create.
While only one-third of American households have a garage, the fueling infrastructure challenges begin in those neighborhoods for an all-EV world.[129] If all garage owners use home chargers, it will necessitate massive upgrades to residential electric networks. Otherwise, as one study showed, “more than 95 percent of residential transformers would be overloaded,” meaning that they fail or can blow up.[130] And there’s also an inconvenient feature in the physics of heat. Even if an EV is fueled at night, when there’s less demand on the grid, the air-cooled transformers (on the local utility poles) can remain overheated after sunrise, when normal residential peak loads begin to heat them up further, thus still increasing the prospects for daytime overload failures, even using off-peak charging.
And for the rest of the non-garage-owning world, the usual mantra of “range anxiety” as an impediment to widespread EV adoption is not true, since most EVs offer gasoline-equivalent range. The key to operational parity with conventional cars is met only with convenient, ubiquitous on-road fast charging.
Shifting the primary energy for mobility from liquids to electrons sounds efficient, but it constitutes a degradation in convenience and an increase in costs for delivering energy. Counterintuitively, at big energy levels, transporting a unit of electrical energy using wires and transformers is about 20-fold more expensive than transporting the same quantity of energy as oil in pipelines and tanks.[131] That gap remains wide, even adjusted for the fact that one-half to one-third as much energy is transported to EVs because of the higher efficiency of electric motors over engines. And the math for convenient electric fueling economics makes it worse.
Overnight fueling uses relatively inexpensive (~$3,000) chargers operating at a 7 kW–19 kW power level.[132] (For comparison, ~3 kW is the power demand for an entire average home.) To approach the convenience of filling a liquid fuel tank in a few minutes, superchargers offer fill-up times of 20–40 minutes by operating at power levels of 300 kW–1,000 kW.[133] Such superchargers cost about $400,000, or more, versus a comparable gasoline pump at under $150,000.[134]
“Fast” charging is still far slower than the five to 10 minutes typical for gasoline fueling. Thus, to achieve the same convenience (avoiding waits in lines, etc.), a filling station will require about four chargers to replace each gasoline pump.[135] Quite aside from the land-use implications, this translates into at least a doubling of the overall cost to build the average filling station (counting land, buildings, and other infrastructures as well).[136] For those who think that “Big Oil” can afford that, we note that only 1% of filling stations are owned by oil companies; nearly all are owned by small businesses.[137]
In addition, installing two dozen or more superchargers at a filling station creates a grid power demand comparable to a small town or a steel mill instead of a convenience-store demand level of today’s filling stations.[138] At the same time, the higher power levels from EV chargers will radically decrease the life span of the existing power transformers on utility poles, coming at a time when costs for new transformers have inflated about fivefold.[139] Moreover, the bigger, heavier transformers needed to support high-power demands will require the replacement of many of today’s 180 million roadside utility poles.
The extra utility infrastructure costs for all that are usually paid by the power user, i.e., the station owner. All these costs will necessarily be added to the fill-up price that consumers pay. In Europe, where there’s more experience with on-road fast charging, the cost of a fast-charge fill-up is already higher than diesel for the same distances driven.[140] In the U.S., Consumer Reports notes that the cost to fill up with a Tesla supercharger is over triple the (usually assumed) cost of at-home overnight charging.[141] Surveys already show that only a small percentage of consumers say that they would be willing to pay the premium for on-road fast charging.[142] But the costs are real and will show up somewhere, even if governments engage in shell games.
Some brag that at-home fill-ups evade the road taxes paid today by other drivers. In an all-EV America, however, the $50 billion a year collected in federal and state gasoline taxes to maintain roads will necessarily shift to EV fueling, one way or another.[143]
As for a “big reset” for EV infrastructure based on the 2022 Inflation Reduction Act’s $7.5 billion in subsidies to fund “thousands” of on-road chargers: consider the math. The all-EV future will need to replace most, if not all, of America’s roughly 1 million gasoline pumps located at the existing 145,000 filling stations. That will require over $100 billion of superchargers, not counting the costs of electrical infrastructure upgrades. The learning curve from future high-volume production, as well as new technologies for superchargers, will shave only a little of that staggering sum.[144] None of these underlying economic impacts is changed by rearranging ownership models for filling stations, whether at supermarkets, shopping malls, parking garages, or automakers building their own, following the Tesla example.[145]
The calculus of overall societal costs for an all-EV future also requires including the dramatic expansion of power generation and long-distance transmission. Replacing all the gasoline used in America with electricity would require at least 50% more electricity generation than exists or is planned, along with an even greater increase in electric power distribution.[146] Such power-plant and grid requirements represent multitrillion-dollar levels of spending.[147]
An analysis from the Boston Consulting Group puts the utility grid improvement costs—never mind power generation—at $1,700–$5,800 per EV put on the market.[148] Do the math: that’s $400 billion to over $1 trillion for an all-EV American car fleet. While those costs would be divorced from car prices, it would constitute “sticker shock” for household electricity or tax bills.
Driving an EV for Most People: Exciting and Expensive
As the Consumer Reports chief for auto testing said: “Consumers tell us reliability is one of the most important factors when buying a car.”[149] It is received wisdom among enthusiasts that EVs have lower maintenance and are more reliable because they’re “simple” machines. Engineering reliability into any machine is the same and emerges from high quality in manufacturing and supply chains.[150] Aside from the earlier-noted fact that EV and ICE complexities are comparable (albeit in different locations), only recently have there been enough EVs on the roads to provide statistically useful reliability evidence with owner surveys and road tests.[151]
In November 2022, Consumer Reports’ annual reliability survey found that of the 11 EV models included, seven rated below average for all cars.[152] Similarly, a UK survey found that during the first several years of ownership, EV owners experienced a 50% higher rate of problems, compared with conventional cars.[153] Notably, problems with software topped the list of quality-control issues for EVs, a feature that is more complex than in an ICE vehicle. Lower reliability invariably shows up somewhere in the overall economics for consumers, even if absorbed by automakers in warranties. To be sure, time will bring reliability equivalence for EVs. One recent three-year study found that overall maintenance and repair costs revealed only “a tiny difference.”[154] Another study, from Car and Driver, found a slightly lower EV maintenance cost.[155] But so far, real-world experience doesn’t seem like a slam-dunk for radically superior EV reliability.
As for insurance, the battery is the most expensive single part of an EV and, typically, costs twice as much as an ICE vehicle.[156] This factor alone leads to higher insurance costs.[157] It is exacerbated by the fact that EV batteries are largely non-repairable and thus become a write-off in the event of even small accidental damage (unlike engine damage, which is frequently repairable).[158]
All the day-to-day operational factors put together determine the real-world costs, as all car owners know. If one includes the high cost of on-road fast charging and, say, assumes lower maintenance and equal insurance costs, the overall cost of ownership for an EV is, according to one deep analysis, some 25% higher than for a conventional car.[159] That cost penalty increases if one attaches an imputed value to the extra time taken for on-road charging (even with fast chargers). This is the kind of cost that economists know influences consumer decisions because people naturally value their time.
Meanwhile, in a price-inflating feedback loop, a radical expansion in grid distribution hardware comes at a time of intense competition for the two key metals—copper and aluminum—needed to build transmission. Those metals constitute 20% of capital costs for electric grids—and that’s before the looming minerals inflation.[160]
Battery waste disposal should be added to the column of unknowns for future EV costs. The unique character, variety, and volume of EV batteries will present recycling and disposal challenges, which have led to rampant speculation about what those costs will be. A Volkswagen-led consortium was launched in 2022 to study the challenges, not to build anything.[161] As the CEO of the nonprofit Call2Recycle said, “Recycling [EV batteries] is not going to be profitable for everybody. That’s fantasy economics.”[162] Whatever the costs, they will ultimately be bolted on to the purchase price of a vehicle, imposed on manufacturers, or subsidized by taxpayers.[163]
A Word About Parity in Supply Chains, Geopolitics, and the Environment
Another favored refrain from EV and green-tech advocates is that the subsidies will free us from “our geopolitical adversaries’ . . . manipulation of the price of oil.”[164] By now, most people following the energy transition narrative are familiar with the deep dependencies on minerals that are produced elsewhere, and they understand that the same geopolitical forces are in play and, indeed, have features that are arguably more worrisome—or at least that they present significant unknowns about future risks.
There is far more supplier concentration for “energy minerals” than for hydrocarbons. Chile, the largest copper producer, with a 20% market share, has a new socialist president who has promised “social justice” and environmental “reforms” in mining.[165] The number-two and number-three producers are Peru and the Congo. Russia, at 10% of global nickel production, is number three in the world and is also a major global supplier of copper and aluminum, at about 4% and 6%, respectively.[166] And China, per IEA, has a “share of refining [that] is around 35% for nickel, 50%–70% for lithium and cobalt, and nearly 90% for rare earth elements”[167] (Figure 14).
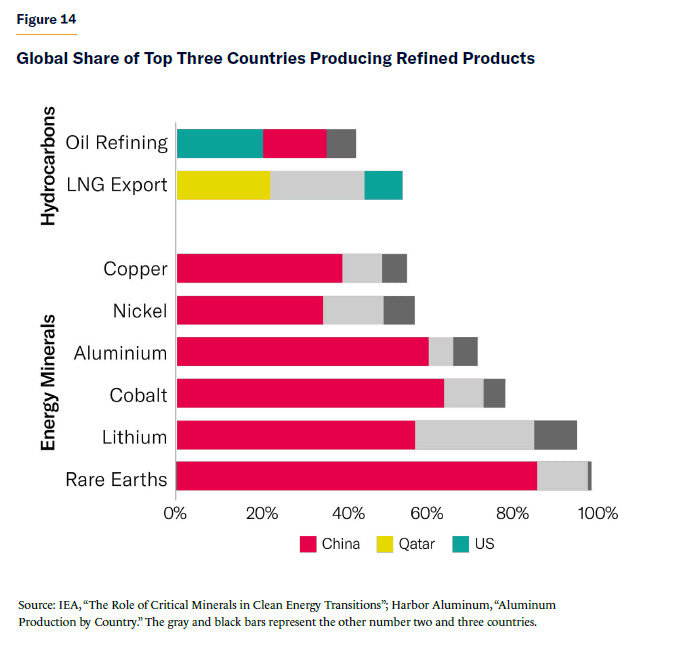
The U.S. today is dependent on imports for 100% of some 17 critical minerals, and, for 28 others, net imports account for more than half of existing domestic demand.[168] Assembling batteries (or solar hardware) here creates underlying dependencies equivalent to assembling conventional automobiles domestically but importing all the key parts and all the fuel.
Hence, we see media accounts and policymakers publicly handwringing over geopolitical mineral dependencies in a fashion redolent of petroleum dependency worries of the past half-century. Dependency anxieties this time around are in advance of anything equivalent to the Arab oil embargo of 1973–74, but the actions aren’t different.
Also, as global mining expands, only now appearing on the radar is a belated recognition of the lack of parity in the environmental challenges from energy minerals, compared with those associated with drilling for oil and gas. As a recent Washington Post investigation noticed, there is a “hidden toll” from “clean cars.” The Post’s investigators visited Indonesia, the world’s top nickel miner. The paper reported that “extracting [nickel], refining it and readying it for export is a gargantuan task” and is “posing steep environmental costs that have yet to be reckoned with.”[169] In another story, the Post’s headline reveals the trend: “The Underbelly of Electric Cars.”[170] Australia’s Institute for Sustainable Futures noted that a global gold rush for energy minerals will take miners into “some remote wilderness areas [that] have maintained high biodiversity because they haven’t yet been disturbed.”[171]
Credit IEA researchers for acknowledging these issues, even if the implications remain largely absent from that agency’s enthusiastic promotion of EVs.[172] Per IEA analysts, mining “can displace communities and threaten natural habitats.” A satellite analysis (necessary because many mines are in uncooperative regions) documented the additional land being ‘consumed’ to expand copper mining. The magnitude of copper alone needed to build forecasted ‘green’ machinery will lead to the use of hundreds of thousands of acres of previously untouched land.
Even less attention has been afforded to the asymmetries in social and humanitarian implications of a massive global shift to energy minerals. As one recent study showed, over half of all the locations where new mining will be needed are lands where indigenous people live that are often ecologically fragile.[173] Northwestern University’s Jennifer Dunn, a pioneer in analyzing life-cycle social impacts, has noted that “technologies that are designed to solve grand challenges such as climate change must consider both their environmental and social impacts to understand their true consequences.”[174]
As environmental researchers in Spain observed regarding the “relocation” of environmental impacts from EVs: “Transfer of environmental burdens from the use phase to the raw materials extraction and manufacturing phases entails a delocalization of the impacts, which constitutes a new challenge at environmental, social, and legal levels.”[175] The researchers estimated that an EV operated in Spain would cut CO2 emissions in half (though, we note, based on an EV with a battery half the popular size) but “will produce an increase in fine particulate matter formation (26%), human carcinogenic (20%) and non-carcinogenic toxicity (61%), terrestrial ecotoxicity (31%), freshwater ecotoxicity (39%), and marine ecotoxicity (41%) relative to petrol vehicles.”
When it comes to environmental parity, the monomaniacal pursuit of CO2 reductions has sidelined the entire range of air, water, biodiversity, and ecosystem protections that were once core environmental goals.[176] It’s a bad trade, not least because EV mandates will lead to trivial, even nonexistent, reductions in CO2 emissions.
Companies representing 40% of global car sales have joined a Science Based Targets initiative to define a “common framework” around automobile emissions, including indirect emissions.[177] Let’s hope that they do follow the science.
Finally, a Word About Behavior Modifications
Given the realities of mineral supplies and uncertainties about associated emissions, IEA and net zero planners have made it clear that “behavior change is critical” to achieving climate goals.[178] For example, “demand side measures such as limiting the growth of battery size” in electric cars can “help bridge the [mineral supply] gap.”[179] The most popular EVs (outside of China) have big batteries to provide the range that consumers want and that manufacturers tout, and because global trends show that buyers want large SUVs—the global SUV share is up from 15% of all new vehicles two decades ago to one-third now, and over one-half in the United States. But according to IEA, “this trend could be curbed by enacting policies that discourage vehicles with extremely large batteries, for example by linking incentives to battery sizes or, in the longer term, taxing EVs with large batteries.”[180] Put differently, that goal is to make it more costly to buy the (larger) EVs that most consumers prefer.
Then, to discourage the use of energy-intensive battery materials and components produced overseas, policymakers promote (and Europe has implemented) the idea of a “border adjustment” carbon tax to induce “cleaner” production and indirectly subsidize domestic sources.[181] While the effect of such policies might lead to slightly more domestic mining and minerals refining, it will certainly raise the cost of batteries and EVs. Certifying who escapes such a tax would require a regulatory apparatus that would dwarf today’s Orwellian automobile fuel-efficiency standards.
That’s not the only behavior change that EV transitionists believe needs to happen. Given the challenges with meeting charging demands, that, too, would be addressed with “behavioral intervention.”[182] Smart-charging will necessarily morph into a regime wherein when and where you can refuel an EV will be controlled, even dictated, by regulators.
Consumers are also to be persuaded, or forced, to drive less in general and travel more by bus, bicycle, rail, ride-share, or on foot, and to own fewer cars in the first place. As stated in the IEA net zero goal: the number of global households without a car needs to rise from 45% today to 70% by 2050, reversing a century-long trend of rising ownership.[183] One researcher simply stated: “There is therefore a need for a wide range of policies that include measures to reduce vehicle ownership and usage.”[184] As usual, California regulators are ahead of the proverbial curve in admitting that the state’s emissions goals will require citizens of that state—on top of being forced into EVs—to drive 25% fewer miles than they did 30 years ago.[185] (emphasis added).
The car culture is viewed in many environmental circles as inherently toxic and unnatural. As a recent New Yorker article put it: “The grip of the car as a metaphor for liberty is as firm as that of guns, if perhaps with similarly destructive results.”[186] The transitionists see car ownership as a “cultural attachment” and profess to believe that “fewer cars on the roads would not mean a sacrifice in the quality of life, convenience.”[187] There would be significant “progress” in keeping people out of cars by banning inexpensive ICE vehicles and mandating expensive EVs.
In the face of all this, it would be reasonable to reach the conclusion that, put simply, they’re coming for your cars.
Conclusion: There’s No Such Thing as a Carbon-Free Lunch
Richard P. Feynman, 1974[188]
Imagining a hypothetical all-EV world requires acknowledging the unavoidable fact of a rats’ nest of assumptions, guesses, and ambiguities regarding emissions. Much of the necessary data may never be collectible in any normal regulatory fashion, given the technical uncertainties and the variety and opacity of geographic factors, as well as the proprietary nature of many of the processes. Those uncertainties could lead to havoc if U.S. and European regulators enshrine “green disclosures” in legally binding ways, and it all will be subject to manipulation, if not fraud.[189]
If ICE prohibitions do take effect, it will happen before EVs are available at a price that most people can afford or have features that most people need or want. One predictable consequence will be far fewer new cars available, leading to a massive increase in the demand for, and the cost of, used ICE cars.
If the policy goal is to reduce automotive petroleum use, there are far easier and more certain ways to achieve that. Combustion engines have already been built and are commercially viable that can cut fuel use by 50%.[190] In fact, an earlier IEA analysis finds that gains in automobile fuel efficiency will displace at least 300% more petroleum than adding 300 million EVs to the world’s roads by 2040.[191]
It would be easier, cheaper, faster—and transparently verifiable—to incentivize consumers to purchase more efficient internal combustion engines or hybrids. Subsidies redirected away from wealthy EV owners would buy far greater, and documentable, emissions reductions per dollar if offered to, for example, lower-income gasoline “superusers”—the 10% of drivers consuming one-third of all gasoline[192]—with a credit tied to trailing odometer mileage. Such a policy would, additionally, be progressive, rather than regressive, in tax terms.
The future will see tens of millions more EVs on the roads, even without government programs that favor or mandate them. But the entire edifice of subsidies, prohibitions, and regulations to move most, if not all, citizens from ICE cars into EVs is based on a profoundly weak—or, in some cases, false—foundation of claims about emissions reductions and economic parity.
Meanwhile, if implemented, ICE bans will lead to a massive misallocation of capital in the world’s $4 trillion personal mobility industry.[193] It will also lead to draconian constraints on freedoms and unprecedented impediments to affordable and convenient driving. And it will have little to no impact on global CO2 emissions. In fact, the bans and EV mandates are more likely to cause a net increase in emissions.
About the Author
Mark P. Mills is a Manhattan Institute senior fellow, a faculty fellow at Northwestern University’s engineering school, and a partner in Montrose Lane, an energy-tech venture fund. He is author of the books The Cloud Revolution: How the Convergence of New Technologies Will Unleash the Next Economic Boom and a Roaring 2020s (2021), Digital Cathedrals (2020), Work in The Age of Robots (2018), and The Bottomless Well (2006). He served as chairman and CTO of ICx Technologies, helping to take it public in 2007. Mills served in the Reagan White House Science Office. Before that, he was an experimental physicist and development engineer in microprocessors and fiber optics.
Appendix: Details Underlying Figure 6
Anatomy of CO2 “Guesstimates” and Known Unknowns: Estimated Lifetime EV Emissions Range from 50% Less to 50% More than ICE
To illustrate the uncertainties in estimating EV lifetime CO2 emissions, we use Volvo’s analysis as a baseline because it is thorough and incorporates many, though not all, variables.[194] Per Volvo: “Choice of methodology has significant impact on the total carbon footprint. . . . [C]are should be taken when comparing results from this report with those from other vehicle manufacturers.” The company showed that, compared with an internal combustion engine’s knowable emissions, the estimated EV emissions range from an idealized 50% lower to a realistic 8% lower.[195]
While theoretical scenarios allow for estimating lower EV emissions, the outcome is not a fact or a measurement. On the other hand, as illustrated below, if the Volvo analysis is adjusted to include some, but not all, known variabilities (discussed in this report), EV lifetime CO2 emissions can be 15% higher than the baseline ICE car, or if the comparison is with a 30% more fuel-efficient engine, estimated EV lifetime emissions can be more than 50% higher.
Below, we summarize six key known unknowns for four upstream materials features that Volvo estimates cause 18 tons of a total of 25 tons of CO2 emitted to build the EV, and we add two downstream sets of variables for the baseline of the additional 16 tons emitted from vehicle charging. (The outcome is illustrated in Figure 6 of this report.)
- Battery size and refueling anxiety: 18 tons CO2 → 22 tons
EV buyers prefer large batteries, not to address “range anxiety” per se but because that minimizes the frequency of on-road fueling that, even with fast chargers, takes 4x–10x longer than with gasoline. Volvo assumes a 71–78 kWh battery. But 90–100 kWh is common, and, as IEA notes, the trend is up. Bigger batteries mean more materials. Assuming a 25% larger battery than Volvo changes the 18 tons of CO2 from materials and refining to 22 tons.
- Emissions from energy used producing battery materials: 22 tons → 25 tons
Volvo shows nonaluminum minerals contributing two-thirds of upstream CO2 based on energy supplied by the “average global grid.” But most “energy materials” are refined in China with a grid that has 50% higher CO2 per kWh. Assuming half the materials are China-sourced adds three tons to revised factor no. 1 above, thus raising the total to 25 tons.[196]
- Energy/emissions from near-future mining: 25 tons → 34 tons
The unprecedented expansion of mining to meet massive EV minerals demands means that each new ton produced will come from mines with declining ore grades (a long-run geological reality), which increases energy use. Energy per ton of copper mined has doubled in the past decade. Assuming only a 50% increase in CO2 per ton for (nonaluminum) materials adds another nine tons to the adjustment in no. 2 above, raising the upstream total to 34 tons.[197]
- Aluminum sourcing: 34 tons → 36 tons
The Volvo baseline shows six tons of CO2 emissions from aluminum production. Specific manufacturers may source aluminum from low-emissions countries, but China is over 55% of the world’s supply.[198] Producing a ton in China emits 20 tons of CO2.[199] The average EV has 0.5 tons of aluminum and rising.[200] Assuming that half the aluminum comes from China adds two tons to the baseline, raising the total battery embodied CO2 to 36 tons.
- Balance of materials: 36 tons → 43 tons
The Volvo baseline includes 7 tons of CO2 from fabricating the balance of hardware (battery module assembly, electronics, other materials, etc.). We ignore for this illustration the known variabilities for those factors but note, for example, that the 200 extra pounds of non-battery copper used for EV wiring and motors entails wide emissions variabilities. Thus, many EVs manufactured now and in the near future will arrive at a dealer, before the first mile driven, with upstream CO2 emissions totaling at least 43 tons.
After the emissions from supplying upstream materials (44 tons) to build the EV, one then adds the variables in downstream emissions, from fueling the EVs battery.
- Drive on U.S. grids, not the EU grid: downstream 16 tons CO2 → 22 tons
Emissions due to battery charging vary, depending on the electricity used (also the time of day, as discussed elsewhere). Average emissions from all U.S. grids, as well as for many regional grids, are about 40% greater than the EU average.[201] With the contemplated expansion of wind and solar, we assume instead a 35% increase. Thus the 16 tons emitted over 120,000 miles of EU charging (per Volvo analysis) becomes 22 tons in many U.S. states.
- Drive vigorously, or use the heater or air conditioner: 22 tons → 24 tons
Using air conditioning, heat, or vigorous driving increases EV energy use from 10% to 50%. Assume that many users will experience at least a 10% increase in electricity used per mile over the rated efficiency, and the 22 tons in no. 5 above increases to 24 tons of CO2.
The Bottom Line: 43 Tons of Estimated Emissions Rises to 67 Tons
The realistic potential of 43 tons of upstream emissions combined with operating realities of 24 tons of downstream emissions (over a vehicle lifetime) yields a total of 67 tons of EV CO2. This is 15% more than the 59-ton baseline for a comparable gasoline-fueled SUV. Or, assume instead that a consumer purchases an ICE car with 30% better fuel efficiency; that vehicle’s lifetime CO2 emissions drop to 40 tons, which is ~27 tons, or 50% less than many possible EV scenarios.
Endnotes
Photo: gerenme/iStock
Are you interested in supporting the Manhattan Institute’s public-interest research and journalism? As a 501(c)(3) nonprofit, donations in support of MI and its scholars’ work are fully tax-deductible as provided by law (EIN #13-2912529).
"electric" - Google News
July 12, 2023 at 07:35PM
https://ift.tt/QEsw5S7
Electric Vehicles for Everyone? The Impossible Dream - Manhattan Institute
"electric" - Google News
https://ift.tt/tGpk41h
https://ift.tt/3QpI5BP
Bagikan Berita Ini
0 Response to "Electric Vehicles for Everyone? The Impossible Dream - Manhattan Institute"
Post a Comment